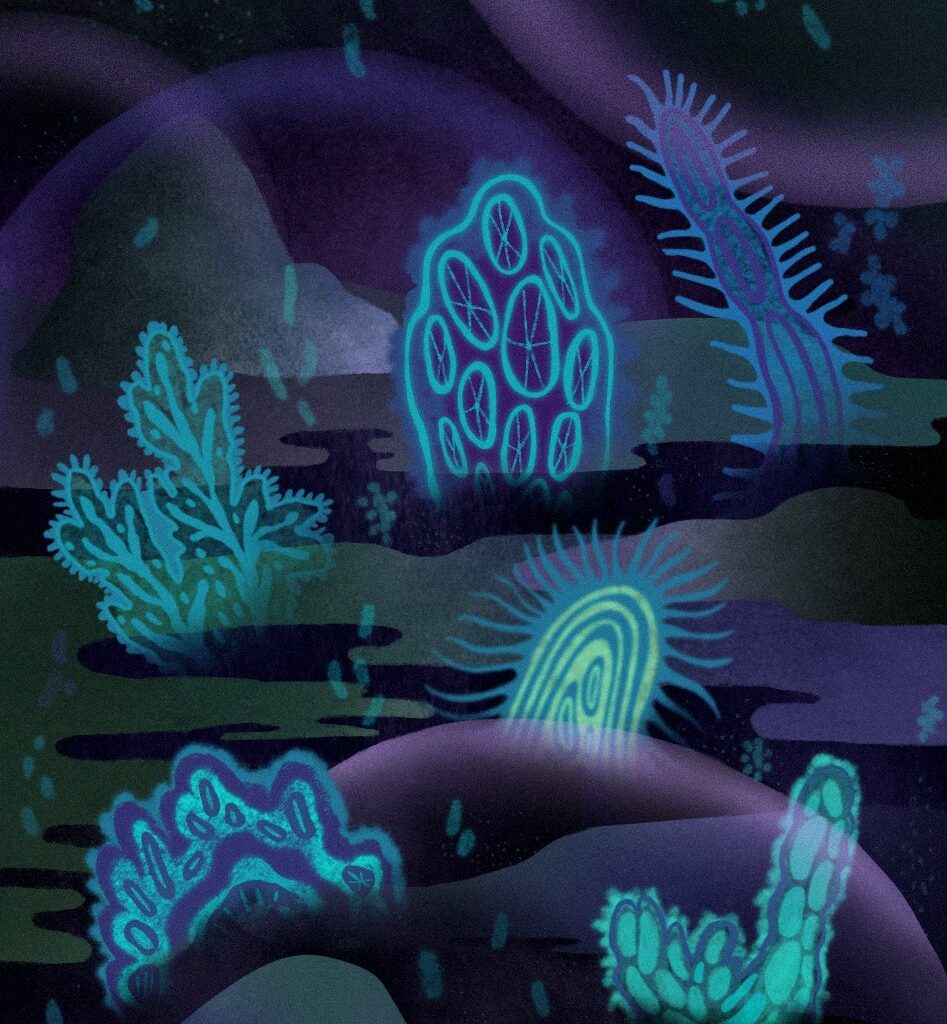
The way to spot a cold methane seep on the ocean floor is to look for the life that gathers around it, like antelopes at a savanna watering hole: clams, mussels, crabs, shrimp, fish, sea anemones and creepy, otherworldly worms. These seeps, exposed by movements of tectonic plates or other geological processes, allow ancient, deeply buried methane to burble through the Earth’s crust and into the water column, where it becomes a kind of manna from heaven — a highly energetic food in what is otherwise a barren desert. Single-celled microbes eat the gas; the crustaceans, worms and other creatures in turn eat the microbes. For microbiologists like myself, this motley crew of creatures is a precious sight, but not because I’m interested in studying them — it’s the tiny microbes I make these half-mile descents for.
Ever since I became a microbiologist, a series of questions has gnawed at me: Are there life-forms hiding inside the Earth? And if there are, how do they survive? Would their nature be so strange that they change our conception of life itself?
The major categories of visible life on Earth have been pretty much settled for centuries. But it wasn’t until the 1980s that scientists found “intraterrestrials” — microscopic organisms living in what the biogeochemist David Valentine calls a “microbial purgatory deep below the Earth’s surface.” Soon followed by other revelations of life inside Earth’s crust, these discoveries revealed that we had been missing major branches on the tree of life. Indeed, these microbes proved that our assumptions about the boundaries of life were wrong — and wildly so.
As it turns out, much of Earth’s habitable space lies deep under thousands of feet of sediments and rock. You might think that any sad little trickle of life in that deep, dark underworld might like to clamber back up to the surface, desperate for lifegiving sunlight. But anyone who’s traveled to a methane seep at the bottom of the sea knows the opposite is true: life flourishes in the dark, and it does so on terms antithetical to those that we who inhabit the thin green layer at the Earth’s surface know.
Intraterrestrials survive without sun and oxygen; instead, via thermodynamics (the art of moving energy around), they are able to respire most elements of the periodic table. Radioactive uranium doesn’t really have a “life support” ring to it, yet that’s just what it is for some intraterrestrials. Arsenic is poisonous to humans and many other creatures, but intraterrestrials respire it too, essentially cleaning up our toxic pollution. Gold is not very chemically reactive — that’s why humans use it in currency and jewelry. Yet there are microbes that consume it.
We know they are alive because we can see the effects of their respiration on the chemicals around them, and we can see that they are intact and not degraded. We know they must be refreshing their cellular biomass. But they are doing it immensely slowly — gradually replacing their parts, lipid by lipid, nucleotide by nucleotide. It takes roughly half a century for them to replace all their molecules.
“Are there life-forms hiding inside the Earth? And if there are, how do they survive? Would their nature be so strange that they change our conception of life itself?”
These single-celled organisms can live for hundreds of thousands, perhaps millions, of years. (By contrast, the oldest multicellular organisms are trees, some of which can live to be thousands of years old, although none of the cells that compose them live for that long.) Theoretically, therefore, when I scoop deep sediment samples, I could be touching microbes that have been living and breathing continuously since well before humans were even a species.
By clinging to the bitter edge of what scientists ever would have guessed is the necessary amount of energy to support life, the intraterrestrials have earned a different relationship with time than we have. Indeed, many endure in environments where they don’t get enough energy to produce new cells, instead surviving on 0.00001% of the power that supports all other known types of cell growth on Earth.
Here’s where the script of life really gets flipped, and where Darwinian evolution needs to be looked at anew. How does a species that doesn’t produce progeny for thousands of years or longer even evolve, given the importance of progeny in the process of evolution?
To answer this question, first we have to think about what these slow organisms would experience in their lifetimes. They aren’t concerned about the length of a day. They’re buried so deep that they can’t detect the sun anyway. They probably don’t even notice a change in seasons. However, they might care about other, longer geological rhythms: the opening and closing of oceanic basins through plate tectonics, the formation and subsidence of new island chains, new fluid flows brought on by the slow formation of cracks in Earth’s crust. The biology I was taught in school considered these events to be evolutionary drivers for a species, not individuals. For instance, Darwin’s finches evolved new beak shapes because they had become isolated on an island with a particular shape of seed to eat. This evolution happened over the geological timescale of island formation, but it occurred in a species lineage, not in an individual bird.
We know, however, that individuals are also capable of changing along with the rhythms of their environment. An arctic fox’s fur changes from white to brown when the snow melts every spring. Many people (not me, sadly) wake up at the same time each morning without the aid of an alarm. Daily and yearly rhythms seem like reasonable things for a person or an animal to keep track of. Ice ages, less so.
Anticipating environmental changes over timescales of eons seems ridiculous. A finch would not evolve the ability to swim because it anticipated that its island would subside into the sea in 100,000 years. But these scenarios may be reasonable for the interstellar travelers. They might count on island subsidence the way humans expect the sun to rise tomorrow.
Buried in the deepest darkness underground, eating strange food, playing with the laws of thermodynamics and living on unrelatably long timescales, intraterrestrials have remained largely remote and aloof from humans. However, the microbes that dwell in the deep subsurface biosphere affect our lives in innumerable ways.
On a planetary scale, they play a key role in regulating Earth’s level of oxygenation. In addition, without the nutrients recycled by intraterrestrials in the seafloor, such as iron and nitrogen, phytoplankton would be severely limited in their ability to make oxygen for us. Intraterrestrials are also uniquely suited to detoxify our worst waste by breathing radioactive uranium, arsenic, organic carcinogens and other nasty stuff. So in effect, they have helped us develop as a species without poisoning ourselves.
Given how intrinsically entwined intraterrestrials are in Earth systems, they might also play an outsized role in how Earth responds to human-made climate change. Each time I climb inside a submersible and sink to the ocean floor or stick a probe into volcanic rock or drill into solid Arctic permafrost, I’m looking both backward into deep geologic history and forward into the future in search of evidence as to how great an impact intraterrestrials could have on our warming planet.
Some of the oldest permafrost in the world is in Siberia, where soils have been frozen solid for at least 1.1 million years. All signs point to the existence of living microbes there — their DNA is intact, not broken to bits like decayed body parts, and they have genes adapted to survive in the thin brine veins that run through permafrost. Much of the carbon on Earth’s surface is bound up in permafrost, so when it thaws, subsurface microbes might increase their metabolic activity, which would convert soil carbon to methane and carbon dioxide, both of which can exacerbate climate change. Almost every summer, new patches of previously frozen ground thaw, waking up new layers of microbes.
“It wasn’t until the 1980s that scientists found ‘intraterrestrials’ — microscopic organisms living in what the biogeochemist David Valentine calls a ‘microbial purgatory deep below the Earth’s surface.’”
Currently, about 1,600 petagrams of carbon are sequestered in permanently frozen soils in Siberia, Greenland, Canada, Alaska, Antarctica, high-altitude locations at lower latitudes and even underwater. This number is about twice the amount of carbon currently in the atmosphere. Turning it all into gas would be catastrophic.
Most global climate change scenario models do not consider the influence of permafrost intraterrestrials. It’s not that the modelers haven’t heard of permafrost microbes or that they don’t think they’re important. It’s that global climate models are not yet able to handle that level of nuance. That’s why I go out in the Arctic to struggle with flimsy drill rods in gusty winds and temperatures around -60 degrees Fahrenheit: to figure out not just how intraterrestrials withstand such harsh conditions, but also whether they are likely to contribute to our doom or perhaps take some of the sting out of climate change.
We do know that some types of microbes hold promise, a possible source of salvation. Microbes in deep aquifers and tiny rock fractures could play a role in a process called carbon capture and storage (CCS), which would remove some of the greenhouse gases from our atmosphere. Most of the excess greenhouse gases currently in our atmosphere came from burning fossil fuels that originated underground. CCS would return those gases underground, but for it to be successful, it has to be really deep — safely out of reach of the atmosphere for thousands of years.
If the subsurface were like an inert container, we could just pump that gas down there, sit back and watch the global temperature stabilize and the glaciers creep back to where they’re supposed to be. But Earth’s crust is not an empty vessel. In addition to all the chemicals in the rocks that might interact with the injected gases, mercurial intraterrestrials are also hiding in every crack and crevice.
Those microbes might suck up this extra carbon from CCS and help turn it into solid rock, making sure it stays well away from our atmosphere for a very long time. Or they might use hydrogen generated from rocks and combine it with carbon dioxide to make methane, which could escape and accelerate global warming. The science of intraterrestrials is far too young to make any clear predictions just yet.
Of course, CCS becomes less critical if we are able to quickly shift away from fossil fuel to energy captured from the sun, wind, tides, temperature gradients, dams, plants and algae. And if those renewables stand a chance of one day replacing fossil fuels, their transport and storage must be flexible; flexibility requires good batteries, and good batteries need metals.
Intraterrestrials have an intimate relationship with a wide variety of metals, many of which are commercially relevant. When intraterrestrials breathe these metals, their respiration often changes the metals’ mineral forms and determines whether they stay sequestered in soil and rock or whether they leach out of it.
Currently, all the metals in the batteries that power our personal electronics (as well as the large lithium-ion batteries used for transportation and high-powered magnets for windmills, for example) are mined. However, a lot of Earth’s metals can be found in grapefruit-sized nodules at the bottom of the ocean and in metal-rich deposits near hydrothermal vents. The process by which these metals accumulate in this way is extremely slow: It takes about a million years to create a layer of metals on a nodule that is as thick as a layer of nail polish. In other words, these polymetallic nodules are not a renewable resource: If someone removed them, it would take longer than the human species has been in existence to detect a noticeable replenishment.
For decades, researchers and entrepreneurs have probed these deep-sea nodules to figure out if they could be profitably extracted. So far, it remains too expensive and difficult. In the past few years, however, precious metals have become increasingly important, drastically changing the economic calculus around deep-sea mining.
Polymetallic nodule mining at a large scale would involve ships lowering dredges the size of a couple trucks onto the seafloor, which then move along it, scooping up everything in the upper few inches. A lot of important biological and chemical processes happen in those upper layers, and nutrients from there travel to the surface ocean, stimulating photosynthesis, fueling fish populations and regulating the gases in our atmosphere.
Deep-sea mining would destroy these layers and much more. After the material dredged from the ocean floor is funneled back up to surface, polymetallic nodules will be separated from sediments that, if dumped overboard, would darken the upper ocean, harming marine animals and killing off phytoplankton, which sequesters greenhouse gases and makes the oxygen and food that support the abundant life in our oceans. Piping it back down to the seafloor would mitigate some of the harms, but there is no consensus on how deep the mining companies should go to release sediment.
“As it turns out, much of Earth’s habitable space lies deep under thousands of feet of sediments and rock.”
The amount of seafloor currently available for nodule exploration is more than four times larger than all the seafloor impacted by offshore oil and gas, and at least twice as large as what is currently dredged for bottom fisheries. The Clarion Clipperton Zone, where companies are currently conducting “small-scale” mining operations, is bigger than India and Pakistan combined. The entire footprint of current mining on land represents only about 3% of the area that may be covered by deep-sea mining if operations are scaled. As a result, hazards are going to pile up, with significant negative environmental consequences for the deep sea.
Polymetallic nodules are not the only targets for commercial mining in the deep sea. Polymetallic sulfides, otherwise known as hydrothermal vents, and cobalt-rich ferromanganese crusts are in the crosshairs too. Hydrothermal vents form when hot, metal-laden subsurface fluids shoot from the deep like a firehose into cold, oxygenated seawater, which forces metals in the fluids to precipitate.
Cobalt-rich ferromanganese crusts, by contrast, form in the areas of the seafloor where ocean currents have swept the area clean of any sediments. These currents expose basalt produced at mid-ocean spreading ridges, which can precipitate metals like cobalt, nickel, platinum, manganese, thallium and tellurium out of seawater. Both of these types of structures make good physical substrates for deep-sea mussels and clams, as well as provide metals that fuel microbial life in the ocean. Like polymetallic nodules, these ecosystems are quietly humming along at the seafloor, providing services that keep the whole ocean in proper balance.
Developing a deep-sea mining industry could wipe away towering spires of minerals built by microbial chemical reactions of deep, ancient fluids. It might destroy unknown intraterrestrials before we have a chance to discover them. Many of the geological, chemical, physical and biological processes happening right now in the deep sea — processes that have been occurring for millions or even billions of years — have yet to be documented. These could also be destroyed, and along with them, the life-giving functions they provide.
The deep sea is one of the least understood places on the planet. I love it because it sits at the sweet spot between mysteriousness and accessibility. But it is imperiled by growing demands for its bounty. Intraterrestrials — almost certainly the oldest life on Earth — can tell us much about how life and Earth co-evolved and the ecosystems that might carry genes or processes that are helpful for medicine or ameliorating climate change.
We only discovered these beings a few decades ago. So far, what we’ve learned about them is mind-blowing. And we learn more every day. Continuing to study them opens up pathways to understanding ways of living that seem completely alien to the life that exists on the surface of the Earth, as well as possibilities for existing in broader harmony with our planet.